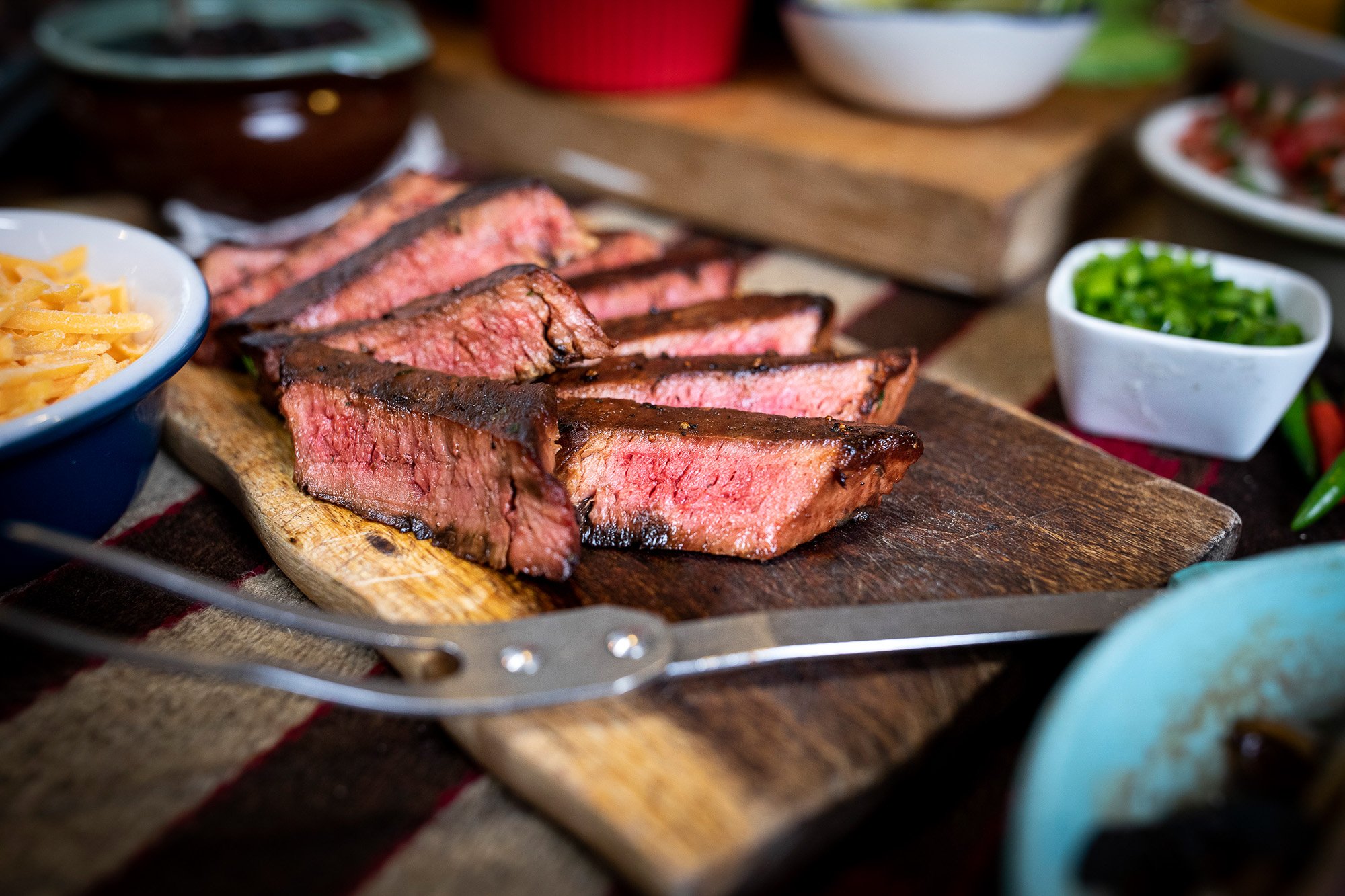
The science of fermentation
Learn about the emerging role of microbial fermentation in building the next generation of alternative protein products.
Tiny organisms, big potential
Fermentation has been used in food production for millennia. Ancient civilizations used microbial cultures to preserve foods, create alcoholic beverages, and improve the nutritional value and bioavailability of foods ranging from kimchi to tempeh. Over the past century, the role of fermentation has expanded far beyond its historical usage to a much broader range of applications.
Fermentation now spans industrial chemistry, biomaterials, therapeutics and medicine, fuels, and advanced food ingredients. The suite of tools developed through fermentation’s evolution is now poised to revolutionize the food sector by accelerating the rise of alternative proteins.
The term “fermentation” carries distinct meanings across different disciplines. Within biology, it refers to a specific metabolic pathway used to generate energy in the absence of oxygen. Within the alternative protein industry, fermentation is used in three primary ways:
Traditional fermentation uses intact live microorganisms to modulate and process plant-derived ingredients.
Traditional fermentation results in products with unique flavor and nutritional profiles and modified texture. Examples are using the fungus Rhizopus to ferment soybeans into tempeh, as well as using various lactic acid bacteria to produce cheese and yogurt. There are also more modern renditions of this concept, such as MycoTechnology’s fermentation of plant-based proteins to improve flavor and functionality.
Biomass fermentation leverages the fast growth and high protein content of many microorganisms to efficiently produce large quantities of protein.
The microbial biomass itself can serve as an ingredient, with the cells intact or minimally processed — for example, the cells can be broken open to improve digestibility or enrich for even higher protein content.
This biomass serves as the main ingredient of a food product or as one of several primary ingredients in a blend. Examples of biomass fermentation are Quorn’s and Meati’s use of filamentous fungi as the base for their products.
Precision fermentation uses microbial hosts as “cell factories” for producing specific functional ingredients.
These ingredients typically require greater purity than the primary protein ingredients and are incorporated at much lower levels. These functional ingredients can improve sensory characteristics and functional attributes of plant-based products or cultivated meat.
Precision fermentation can produce enzymes, flavoring agents, vitamins, natural pigments, and fats. Examples include Perfect Day’s dairy proteins, Clara Foods’ egg proteins, and Impossible Foods’ heme protein.
Innovations are occurring across all three types of fermentation.
Despite microbial fermentation’s long history in food and industrial biotechnology, tremendous potential for innovation remains untapped. The vast biological diversity of microbial species, coupled with virtually limitless biological synthesis capabilities, translates to immense opportunity for novel alternative protein solutions to emerge from fermentation-based approaches.
Opportunities for advancing fermentation can be segmented into five key areas spanning the value chain: target selection and design, strain development, feedstock optimization, bioprocess design, and end-product formulation and manufacturing.
Target selection and design
When microorganisms are used as production hosts to create specific high-value ingredients, identifying and designing the right target molecules to manufacture is key.
The current state of target selection
Biology provides food developers with an almost boundless palette of molecules from which to assemble flavors, textures, and aromas. However, not all these ingredients are easily sourced at large volumes and low prices. By using microbial cells as the production host, precision fermentation allows for highly scalable manufacture of virtually any ingredient.
Target selection and design is the starting point for the process of precision fermentation. The molecule or molecules of interest are referred to as the target. The target can be a protein, a lipid, a flavor compound, a fragrance, an enzyme, a growth factor, a pigment, or another class of molecule.
Fermentation-derived ingredients are already widely used across the food industry.
The majority of vitamins in nutritional supplements and fortified processed foods, such as B12 and riboflavin, are produced through fermentation, as are many flavoring components. The food industry was among the first to leverage fermentation to displace animal products in everyday use.
Commercialization of fermentation-produced chymosin (the major enzyme in calf rennet, taken from the lining of calves’ stomachs) in the 1980s rendered calf rennet’s previously vital use as a coagulant in cheesemaking obsolete for most global cheese production.
A notable recent example of precision fermentation’s use in alternative proteins is Impossible Foods’ use of purified soy leghemoglobin. “Heme,” produced using Pichia pastoris, is used as a flavoring ingredient in their burger to produce a suite of organoleptic properties in the cooked product.
Other recombinant proteins, such as casein and whey, are key targets because of their unique functionality in dairy products. These proteins can be combined with plant-derived ingredients to create a final product. For example, sugar, coconut oil, and sunflower oil are combined with fermentation-produced recombinant whey to make Perfect Day’s ice cream base.
Precision fermentation targets specific molecules.
Target molecules such as animal-origin-free growth factors are used in the production of cultivated meat. Several companies, including ORF Genetics, Richcore, and Peprotech, already work in this space. Furthermore, proteins such as collagen or fibronectin produced through fermentation may serve as key animal-free components of scaffolding for more complex, highly-structured cultivated meat products.
In the case of a protein target, the instruction manual for synthesizing the protein is encoded in the host organism’s DNA, either as a naturally occurring gene or as a gene introduced through engineering. Depending on the target, both engineered and non-engineered approaches may be possible.
For example, the soy leghemoglobin protein produced by Impossible Foods is engineered into a yeast host strain for efficient, scalable production. On the other hand, microalgae company Triton Algae Innovations is commercializing heme proteins that are native to their algal strains, so no engineering is involved.
Synthesis of non-protein targets cannot be encoded directly in the host’s DNA. Instead, the genome encodes a series of enzymes that compose the biosynthetic pathway for producing the target molecules.
For example, the target molecules for algal omega-3 production are the fatty acids DHA and EPA, but the instruction manual for manufacturing these fatty acids consists of several gene-encoded enzymes that convert precursor fatty acids into these desirable fatty acids within the cell. As with protein targets, molecules like fats or flavoring molecules can be produced in microbial hosts either with or without the use of engineering techniques, depending on the specific target and the choice of host organism.
Challenges in target selection for precision fermentation
One of the most basic challenges for target selection is simply determining which molecules contribute the most to specific properties of animal products. A litany of volatile compounds, many of which differ by species type and cut, contribute to the taste of different kinds of meat. These compounds should be more holistically characterized and cataloged to develop a consensus “wish list” of target molecules as candidates for production through fermentation.
Mass-producing already-existing molecules
In many cases, several variants of a candidate target may already exist in nature. For example, almost every living organism contains heme proteins of some sort, but which ones perform the best as flavor enhancers for meat products? Which are the most stable — not only during their production, but also through the downstream processing of the final food product and throughout its shelf life? Which target accumulates at the highest titers within host cells, thus allowing for the most favorable economics?
All of these answers must be ascertained through a combination of thorough empirical screening and predictive approaches.
For target molecules that are not proteins, there are additional challenges: identifying biosynthetic pathways that can manufacture these molecules, and then determining whether these pathways already exist in suitable host organisms or if they must be engineered or enhanced for higher productivity.
For example, fermentation-derived lipid production is relatively unexplored for food applications but has a fairly robust history for industrial chemicals. The alternative protein industry may be able to develop an open-access research foundation and accelerate the commercialization of fermentation-derived fats by aggregating lipid synthesis pathway insights from the chemicals industry.
Each of these aspects feed into one of the key challenges within precision fermentation: improving the economics of production. To compete with animal-based proteins, researchers and companies must increase the titer (amount of an expressed target molecule relative to the volume of total upstream-produced liquid containing the agent – the primary benchmark of upstream efficiency) and yield (the ratio of the mass of final purified protein relative to its mass at the start of purification – the primary benchmark of downstream efficiency) of target molecules and protein biomass.
While strain development and feedstock optimization can contribute substantially to the overall economics, the target selection is a critical factor in achieving economic viability.
Where target selection innovation for precision fermentation is headed
Fermentation allows for a decoupling of the original source of a target molecule and its production method. This decoupling vastly expands the search landscape for biomolecules with unique and valuable functions.
First, ideal targets may originate in species that are extraordinarily rare, difficult to harvest, expensive, or otherwise inaccessible or impractical. Fermentation provides a mechanism for manufacturing these molecules at scales and prices suitable for commercial viability.
Second, targets are not limited to those found in nature: Novel variants of target molecules can be engineered through random alteration and screening (directed evolution) or through rational design, leading to targets that exceed the performance of any naturally occurring version.
Mastodon collagen anyone?
Geltor’s collagen production platform illustrates both aspects of this expanded search landscape. Gelatin (a form of collagen) from conventional animal sources is limited to a few species (predominantly pig and cow, although fish gelatin is also commercially available) that are processed in large quantities. But collagen is ubiquitous in the animal kingdom, and Geltor can manufacture collagen proteins from any species, including extinct species.
In 2018, the company showcased the versatility of their platform with an animal-free leather binding made from jellyfish collagen and gummy snacks made with mastodon collagen. Geltor also makes bespoke versions of collagen that are precisely tuned to the characteristics desired for a particular application — for example, gelatin that exhibits a specific gelling viscosity, elasticity, or melt temperature.
Similarly, fermentation allows for enzymes to be adapted or engineered to exhibit higher activity, novel substrate specificity, greater stability, or robustness under specific processing conditions, with dramatic implications for cost reduction. Such enzymes serve many purposes across the alternative protein industry.
These examples show that fermentation holds immense potential to screen for natural variants of targets and to design new variants for augmented sensory, functional, or nutritional properties or for attributes that reduce costs and streamline manufacturing processes.
Check out related resources
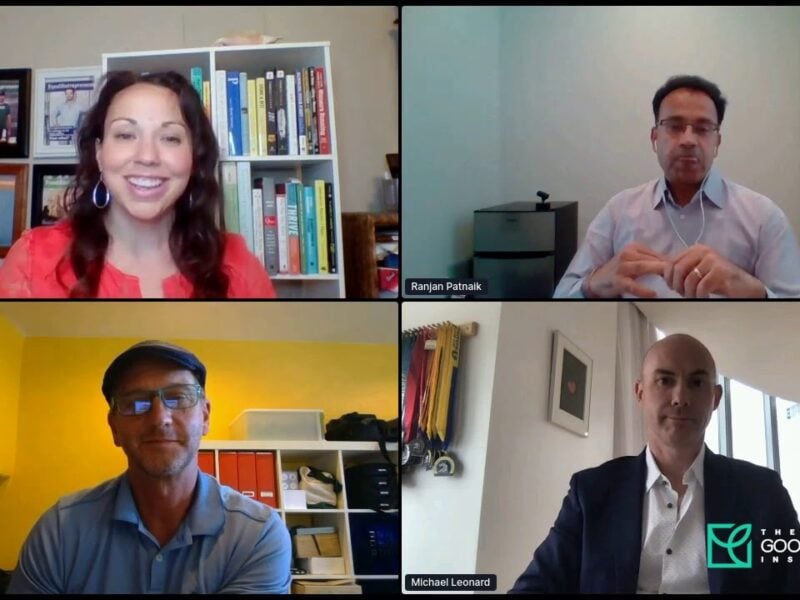
Harnessing microbial hosts as cell factories
Watch industry leaders discuss precision fermentation at GFI’s 2020 Fermentation Symposium.
Rethinking Food and Agriculture by Rethink X
Download Rethink X’s report on how food production will transform by 2030.
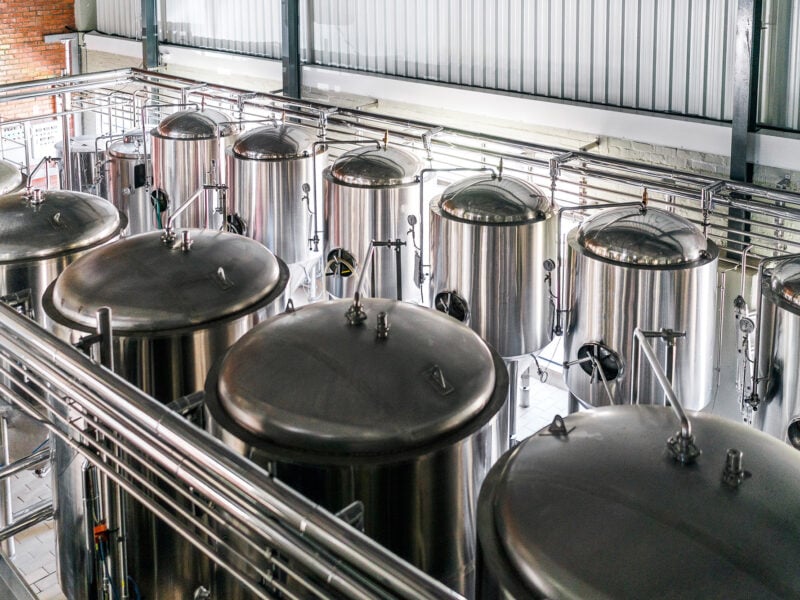
Precision fermentation and cellular agriculture
Learn how cellular agriculture makes it possible to produce genuine animal protein through microbial precision fermentation.
Explore research opportunities in target selection
-
Cultivated
-
Fermentation
-
Plant-Based
Optimizing fat profiles for nutritional and sensory properties
Because alternative meat’s fat content and fatty acid profile can be more easily controlled than conventional meat’s, there is an opportunity to alter fat content for nutritional benefits. Additional research…
-
Fermentation
Novel methods for long-chain omega-3 fatty acid production
As the alternative seafood industry scales up, a low-cost and abundant source of long-chain omega-3 polyunsaturated fatty acids will become necessary. Several means of producing these compounds have been investigated…
-
Cultivated
-
Fermentation
-
Plant-Based
Preventing oxidation of omega-3 fatty acids before and after addition to alternative seafood products
Deeper fundamental knowledge of the causes and prevention of oxidation of omega-3 fatty acids before, during, and after addition to alternative seafood products is needed to improve their nutritional and…
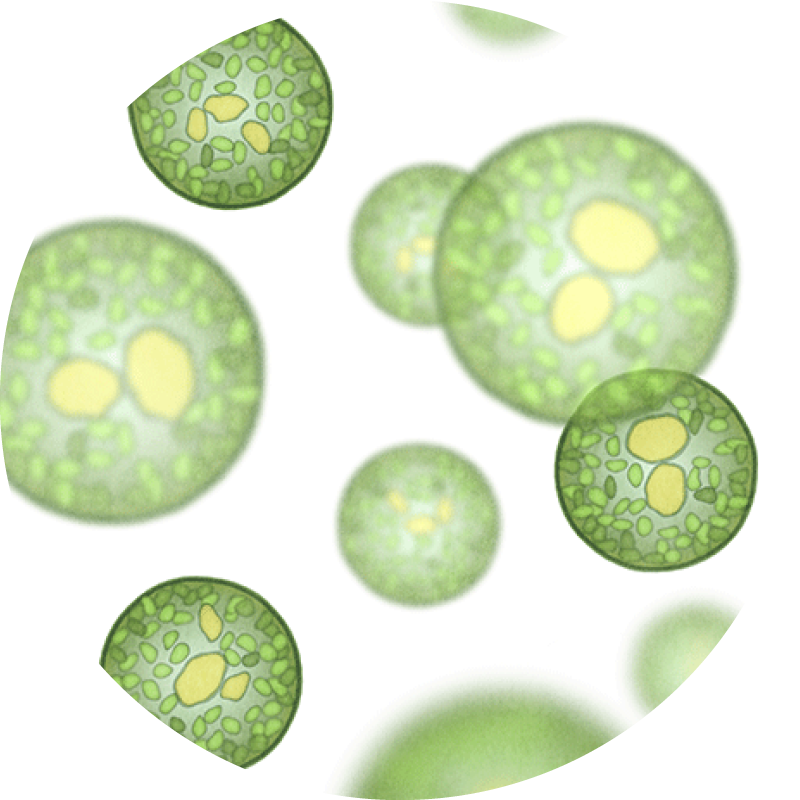
Strain development
Microbial strains offer immense biological diversity, which can be leveraged to identify or create strains with enhanced growth potential, nutritional characteristics, flavor profiles, or feedstock preferences.
Current state of microbial strain development
In theory, microbial fermentation encompasses an enormous variety of species with vast biological diversity, ranging from fungi to bacteria to microalgae. However, exceedingly few microbial species have ever been commercialized for use in food.
New technologies allow us to overhaul the search for new lines.
The emergence of high-throughput screening and characterization tools, in addition to in silico capabilities, merit a recanvassing of all known microbial species for their potential suitability as protein sources.
For example, the strain Fusarium venenatum, the filamentous fungus first commercialized by Quorn, emerged from precisely such a screening effort. This particular search was initiated in the 1960s over concerns about an impending protein shortage, but the constraints of that era’s research tools meant that discovery efforts were limited in scope.
Likewise, the catalog of host strains used as microbial factories for producing high-value targets is also overdue for an overhaul. For decades, the fermentation sector has relied predominantly on a small number of well-established staple species.
While there is room to further design these species for higher yields, more robust cultivation, faster growth, and other ideal production traits, the sector has been limited to these species because of their longstanding use and familiarity, as well as regulatory barriers to commercializing new host species.
Challenges in microbial strain development for fermentation
Comprehensive strain discovery and development programs require massive data sets and specimen libraries to ensure broad capture of sufficient biological diversity, genomic data, and distinct growth conditions. These data sets and libraries — sometimes called biofoundries — are expensive to create and intensive to maintain. Thus, few private companies are capable of committing the time and resources required to create them, and those that do are incentivized to retain proprietary access to justify their investment.
These data limitations restrict and delay commercial adoption of novel or improved strains, and limit access to only certain players. Some publicly-funded biofoundries have been established, such as the Department of Energy-funded Agile BioFoundry and a handful of global efforts within the Global Biofoundries Alliance, but dedicated resources are needed to apply screens for identifying strains suitable for alternative protein applications.
Coordinated strain discovery to accelerate alt protein improvements.
Strain development efforts that are more targeted — such as improvements to existing strains rather than comprehensive screening efforts — are likely to contribute incremental advances but may not achieve the step changes or paradigm shifts in capabilities that the alternative protein field requires. These disaggregated approaches may also fail to generate deeper insights about microbial biology — such as how metabolism can be shifted in desirable ways to enhance productivity, or which genetic signatures indicate suitability for various feedstocks or growth conditions — that could accelerate efforts across multiple strains.
Companies hoping to commercialize novel or significantly altered strains also face regulatory barriers, which present a hurdle to innovation but provide relatively little competitive advantage when addressed in the private sector. Coordinated strain discovery and development efforts that would standardize and streamline the generation of safety data could accelerate more widespread adoption of new strains. This could drive improvements in nutritional quality, production efficiency, sustainability, or even end-product traits like flavor and texture.
The future of strain development for fermentation
A program of systematic strain discovery and development conducted with today’s tools — and bolstered by computational insights based on the wealth of genomic data now available for tens of thousands of microbial species — could yield entirely new candidates for microbial protein production, in addition to providing a roadmap for continued improvement of existing strains.
High-throughput methods of strain selection, adaptation, screening, and engineering enable innovators to iterate new strains with greater speed and precision. They can select for more nuanced attributes, such as precise flavor-enhancing metabolite profiles, rather than simple traits like growth rates or temperature tolerance.
While some of the strain development work in this sector is likely to involve biotechnological tools, such as gene editing and genetic engineering, vast progress remains to be made through simple adaptation and breeding strategies powered by advanced genomic insights.
Clearing the way for the next generation
Strain development research can pave the way for new workhorse strains that can significantly outperform the incumbents. We also need visionary regulatory leadership to streamline commercial adoption of new candidates. Fermentation should not be stymied by historical allegiance to legacy host strains or outdated regulatory procedures.
In addition, comprehensive efforts should be undertaken to assimilate systems biology insights regarding metabolic pathways across species to aid in identification and design of novel hosts with ideal attributes. These attributes may include prolonged generational stability to support long-term continuous culture, metabolic pathways that use a wide range of feedstocks, a desirable flavor profile, and low levels of nucleic acid or other undesirable metabolites.
Explore research opportunities in strain development
-
Fermentation
Novel methods for long-chain omega-3 fatty acid production
As the alternative seafood industry scales up, a low-cost and abundant source of long-chain omega-3 polyunsaturated fatty acids will become necessary. Several means of producing these compounds have been investigated…
-
Cultivated
-
Fermentation
-
Plant-Based
Preventing oxidation of omega-3 fatty acids before and after addition to alternative seafood products
Deeper fundamental knowledge of the causes and prevention of oxidation of omega-3 fatty acids before, during, and after addition to alternative seafood products is needed to improve their nutritional and…
-
Fermentation
Microbial strain-development contract research organizations for alternative protein applications
While emerging fermentation-derived ingredient companies often optimize their strain’s productivity in-house, it may be more efficacious for startups to engage contract research organizations with both deep microbial strain development expertise…
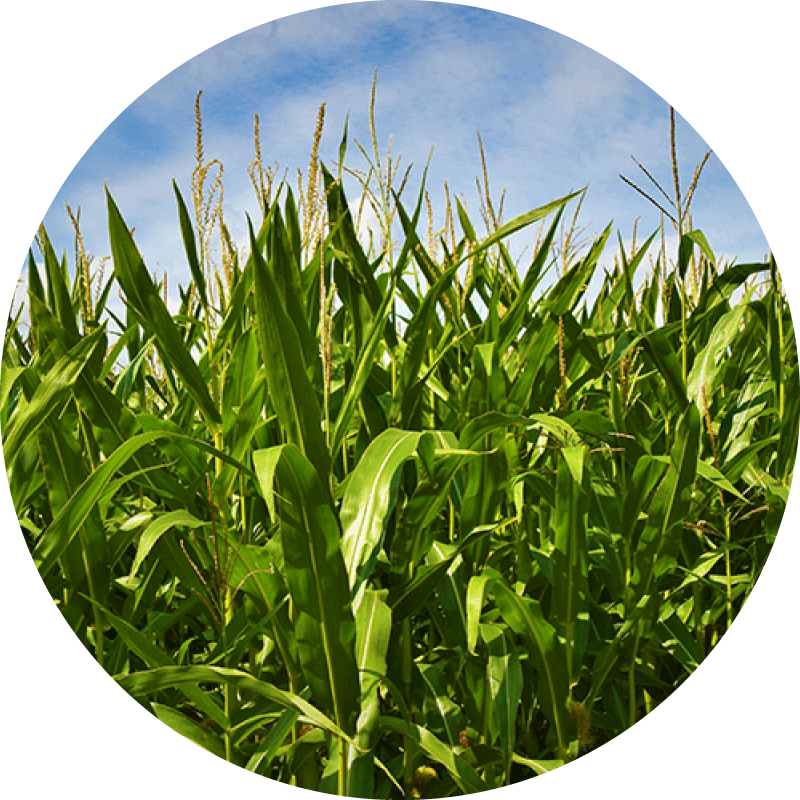
Feedstock optimization
Among the most compelling features of fermentation is the potential to use diverse and malleable feedstocks, such as leveraging existing agricultural sidestreams for economic and sustainability advantages.
The current state of feedstocks for fermentation
Feedstocks provide the nutrients — the basic building blocks of life — to support microorganisms’ growth during fermentation. Much of the resiliency and adaptability of fermentation derives from its innate malleability with regard to these feedstock raw material inputs.
At the same time, feedstocks are a major cost driver for most fermentation processes. Thus, a great deal of optimization is possible in engineering industrial-scale production schemes to use unconventional feedstocks, including potential sidestreams from other industries. This presents potential gains for both economic viability and sustainability.
At present, the majority of fermentation relies on fairly standardized, refined, sugar-based feedstocks. These have a long history of validated use in both food and industrial biotechnology fermentation processes. To reach mass commercialization, cheaper and more sustainable substrates must become widely available. Additional research is needed to move beyond this paradigm and empower fermentation companies to leverage more diverse inputs.
The challenges for optimizing fermentation feedstocks
Due to the sheer volume of raw materials required, feedstock is a key input cost in the fermentation process, regardless of the microbe or downstream processing techniques. Furthermore, shipping costs for feedstocks are high relative to the cost of the feedstock itself.
While these are not notable bottlenecks for current uses of fermentation because sugar feedstocks are sufficiently cheap, of high enough quality, and in large enough supply, growing demand for fermentation will result in substantially increased needs for traditional feedstocks.
Growing demand for fermentation is an opportunity to diversify.
Ultimately, this growing demand may become problematic but also represents an opportunity to diversify.
Alternative feedstocks remain highly inconsistent and poorly characterized. There are concerns around the food safety and regulatory issues that may arise given the use of a lower grade, unconventional input such as an agricultural sidestream.
A shift toward these more diverse feedstocks would be easier if widely-adopted ingredient standards were established and trustworthy, with comprehensive characterization methods easily available. There is a need not just for technological solutions but also market-based solutions in the form of marketplaces, exchange platforms, brokers, and services that can facilitate matching ingredient buyers and sellers. This would include easily ordering R&D quantities and simple comparisons between different suppliers and products.
The future of better feedstocks
An increasing number of companies and researchers are capitalizing on the potential to convert waste products or agro-industrial byproducts into high-quality protein biomass. Nature’s Fynd produces protein from extremophile fungi isolated from a thermal spring in Yellowstone National Park.
These fungi exhibit wide metabolic flexibility and therefore can use diverse feedstocks. 3F Bio and Mycorena in Sweden also position themselves as leaders in sustainable feedstock use. Other startups, including Air Protein, leverage gaseous feedstocks, deriving energy from chemical reactions involving hydrogen, methane, or carbon dioxide gas.
Building a global bioeconomy
Feedstock optimization should be considered in the context of global shifts in demand across many biological raw materials. The rise in demand for fermentation feedstocks is driven by a wholesale shift toward a bioeconomy model of production. This bioeconomy could potentially leverage microbial platforms for manufacturing not just food and pharma products but also green chemical products, biopolymers, and fuels that have historically been dominated by petrochemical-based production.
With this perspective, it is possible to engage in more strategic decision-making regarding the location of new fermentation facilities, placing them near abundant low-cost feedstock sources.
Feedstocks should also be examined across all alternative protein production platforms, including plant-based and cultivated. All these production modalities currently require slightly different feedstocks as primary inputs, and strategic forecasting of raw material demands across all sectors informs better decision-making regarding processing, sourcing, and formulation.
The industry’s ability to nimbly tap into diverse, unconventional feedstocks will also be bolstered by the adoption of globally recognized standards and the development of novel characterization technologies. These will give purchasers confidence in the quality and performance of the feedstock material they buy. These standards will also equip them with the predictive capacity to adapt their process as needed to suit a given lot, even if it is from a source or of a composition they have not routinely used in the past.
Fermentation as the ultimate sustainability solution
Watch industry leaders discuss the sustainability of fermentation at GFI’s 2020 Fermentation Symposium.
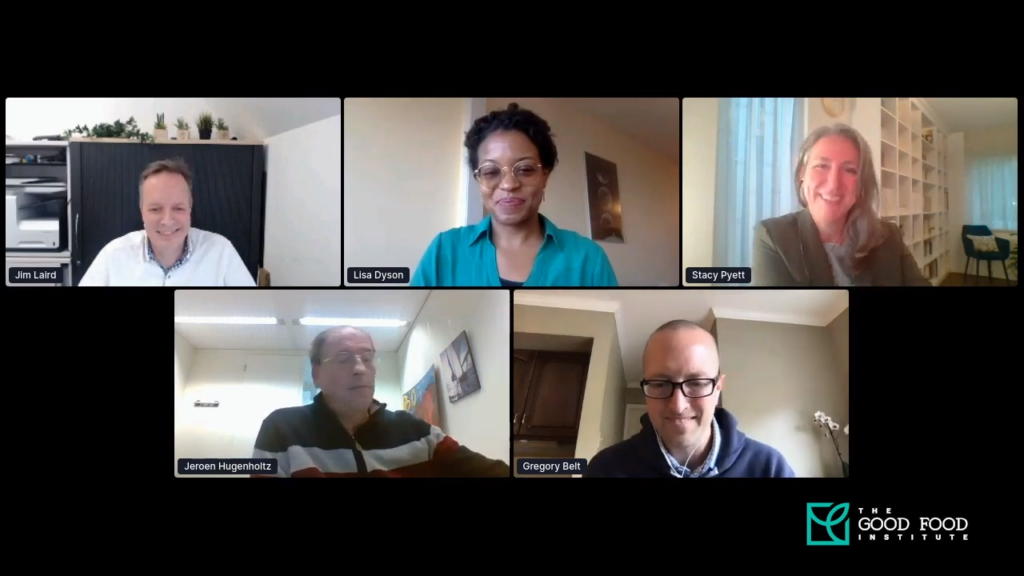
Explore research opportunities in feedstock optimization
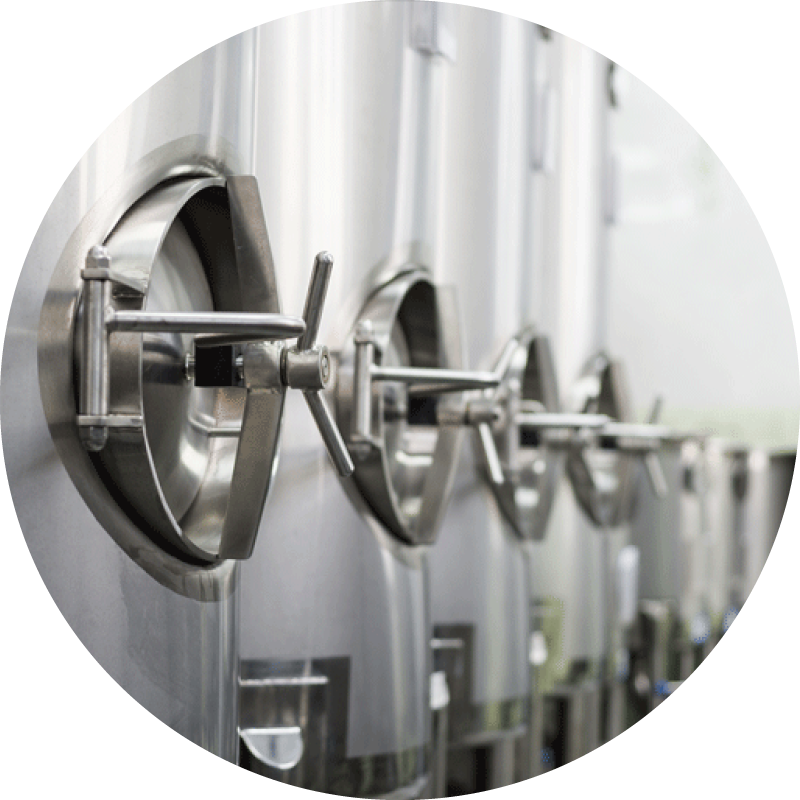
Bioprocess design
Innovations in bioprocess design can unlock new opportunities for cost reduction, scale-up, and environmental sustainability for fermentation’s use within alternative proteins.
The current state of bioprocess design
For decades, industrial microbial fermentation has operated at massive scales, with individual cultivation tanks as large as hundreds of thousands of liters. However, these production facilities represent relatively limited options for process design to accommodate novel organisms or to suit the manufacturing requirements of alternative protein applications of fermentation.
For example, the vast majority of fermentation facilities currently operational for industrial biotechnology and bioethanol production use submerged fermentation, meaning that the microbial cells are suspended in a liquid nutrient medium. However, some of the structured and intact uses of whole-cell biomass (e.g., whole meat cuts) rely on solid-state fermentation, where the microbes are inoculated onto a moistened solid feedstock that may be enclosed or even grown in open air.
Even within submerged fermentation bioprocesses, the scale, cost sensitivity, and sustainability considerations associated with alternative protein applications may warrant approaches distinct from classical stirred-tank bioreactors. Some fermentation companies are successfully using novel bioprocess and bioreactor designs, but relatively little attention has been paid to further optimization or iteration of these designs simply because few companies have used them to date.
Clearly, there is still ample room for innovations in bioprocess design to meet the unique needs of the alternative protein industry.
The challenges for better bioprocess design
Although many current assumptions about fermentation deserve to be challenged, bioprocess innovation can represent substantial risk. Especially in the current environment where fermentation capacity is often limiting — either for companies seeking contract manufacturing partners or for companies seeking to purchase an existing facility — there may be few opportunities to radically alter key aspects of the fermentation bioprocess design. The more the process mirrors what has been done for decades prior, the less risk there will be for subsequent scale-up stages. This makes short-term investment capital easier to come by.
Step change requires breaking from the past.
However, mirroring the past leaves the industry lacking the true game-changing innovations that can ultimately facilitate a step change in productivity, scalability, and cost reduction. Innovations in basic bioreactor design could accommodate denser or more viscous cultures, facilitate nutrient and air exchange at larger volumes and with less energy consumption, or enable longer periods of continuous production. Aspects of the process beyond microbial cultivation can also radically improve efficiencies.
Possible advances include novel harvesting methods and innovative cleaning protocols to reduce equipment downtime and ensure safety. Downstream purification and post-harvest processing requirements will vary widely as well. Current enzyme production processes represent one paradigm, but these processes typically assume very high purity is required, whereas this may be unnecessary for many of the flavoring ingredients or functional proteins used in alternative protein products.
Open-access technoeconomic models for assessing trade-offs between various parameters throughout the production process do not exist for alternative protein applications of fermentation. This precludes more rigorous insights on the most promising focus areas within bioprocess innovation.
What could be next for bioprocess design?
Alternative protein players using fermentation have already demonstrated some bold thinking in bioprocess innovation. For example, Quorn pioneered a bioreactor design called air-lift fermentation, which requires substantially less energy than conventional bioreactors while accommodating large volumes. This bioreactor design is well suited for filamentous fungi, which increase the viscosity of the solution more than non-filamentous fungi or bacteria.
Animal feed companies using gaseous feedstocks — such as Unibio and Calysta, who work with methanotrophic bacteria — often apply a similar concept, whereby feedstock gases and other gases circulate the cells and media in an enclosed loop, eliminating the need for an impeller.
Several companies using fermentation to create whole muscle cuts or to convert plant-based proteins into more functional ingredients are exploring the use of solid-state fermentation platforms. These systems may offer cost savings and lower barriers to entry because they do not require the same capital-intensive stainless-steel bioreactors needed for submerged fermentation.
Solid-state fermentation platforms also open the door to scale-out approaches rather than scale-up approaches — increasing capacity through parallel small-scale units rather than larger-volume single units. This approach mitigates some of the technical risks and capital costs associated with scaling.
Are existing facilities an opportunity?
Another key opportunity area is research into retrofitting existing manufacturing facilities and equipment to suit the needs of alternative protein applications. For example, most existing fermentation infrastructure was built for anaerobic bioethanol production.
As the world moves increasingly toward renewable energy and electrification, these facilities may be decommissioned in the coming decades. However, deeper analysis is needed to understand under what circumstances converting these facilities for alternative protein applications would be technically feasible or fiscally merited.
White Dog Labs recently purchased an ethanol plant with plans to convert it to aquaculture-feed protein production. They can do this because their microorganism tolerates anaerobic growth. This example illustrates the importance of coordinated R&D efforts across all the key technology development areas to capitalize on insights at the interfaces of these disciplines.
Most microorganisms currently used for food ingredient fermentation require aerobic growth, but if technoeconomic and engineering analyses indicate that conversion from bioethanol facilities to anaerobic food production facilities is highly feasible, then anaerobic growth should be a key screening condition within comprehensive strain assessment efforts.
Biomass fermentation: Microorganisms as protein powerhouses
Watch industry leaders discuss biomass fermentation and the incredible efficiency of microorganisms for protein production at GFI’s 2020 Fermentation Symposium.
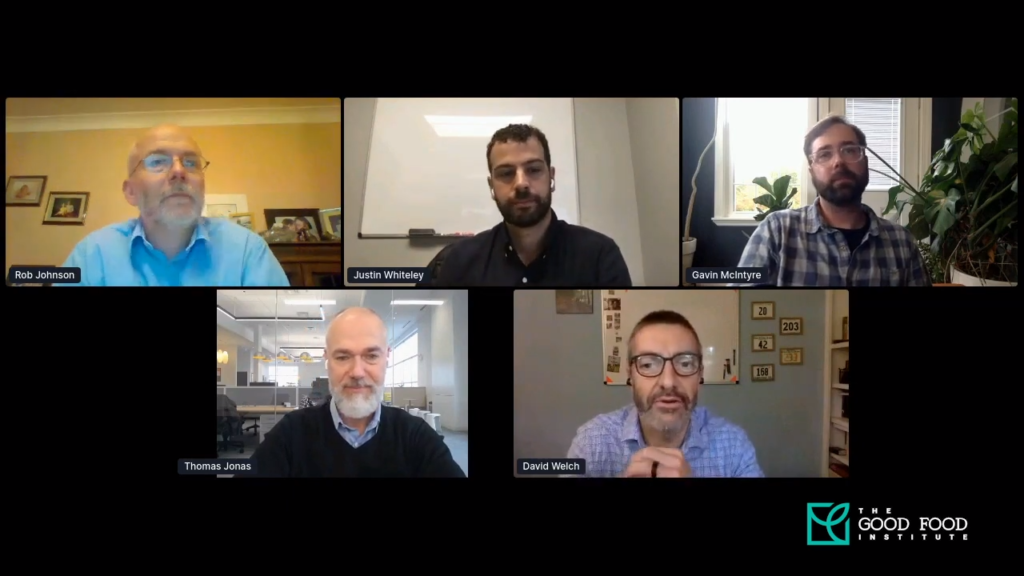
Explore research opportunities in bioprocess design
-
Cultivated
-
Fermentation
-
Plant-Based
Repurposing and retrofitting facilities for use in alternative protein manufacturing
The manufacturing capacity for rapid and cost-effective scale-up of alternative protein production is a current constraint on the growth of the industry. Repurposing and retrofitting stranded or underutilized assets such…
-
Fermentation
Novel methods for long-chain omega-3 fatty acid production
As the alternative seafood industry scales up, a low-cost and abundant source of long-chain omega-3 polyunsaturated fatty acids will become necessary. Several means of producing these compounds have been investigated…
-
Cultivated
-
Fermentation
-
Plant-Based
Preventing oxidation of omega-3 fatty acids before and after addition to alternative seafood products
Deeper fundamental knowledge of the causes and prevention of oxidation of omega-3 fatty acids before, during, and after addition to alternative seafood products is needed to improve their nutritional and…
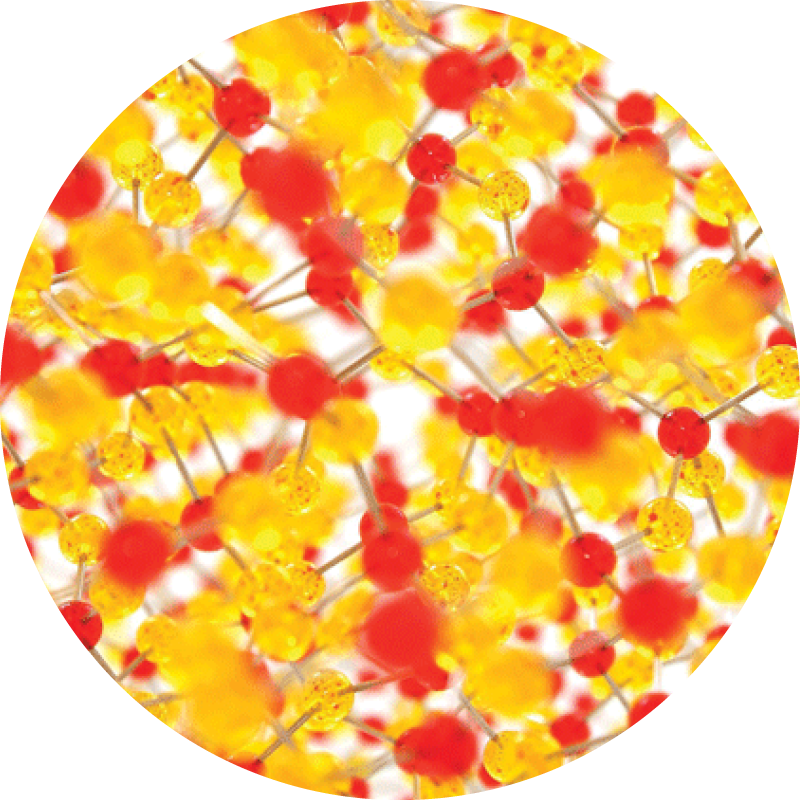
End-product formulation and manufacturing
With fermentation-derived products still an emerging category in alternative proteins, they can achieve even greater sensory and textural breakthroughs through innovations in formulation and manufacturing.
Fermentation companies that make consumer products have the same opportunities for innovation in formulation and manufacturing as plant-based meat companies. In some cases, additional post-harvest processing steps may be required to endow the microbial biomass with the desired structure and texture.
For example, Quorn applies a freezing step to consolidate the delicate mycelial fibers into more durable, aligned bundles that more closely resemble the fibers in animal muscle tissue.
Fermentation-derived ingredients cost less and create better texture.
There is ample room to develop other novel, relatively low-tech and low-cost structuring solutions. These can further improve texture without the capital costs associated with the high-moisture extrusion used to produce many current plant-based proteins.
Individual companies will iteratively improve on their recipes, potentially incorporating flavorings, fats, binders, functional enzymes, and nutritional fortification to achieve sensory profiles that more closely mimic those of their animal-derived counterparts.
In turn, many of these ingredients may themselves be produced by B2B providers of fermentation-derived ingredients. Thus, many opportunities for innovation at the end product level are identical to those of plant-based products.
Leveraging the power of biology through traditional fermentation
Watch industry leaders discuss traditional fermentation at GFI’s 2020 Fermentation Symposium.
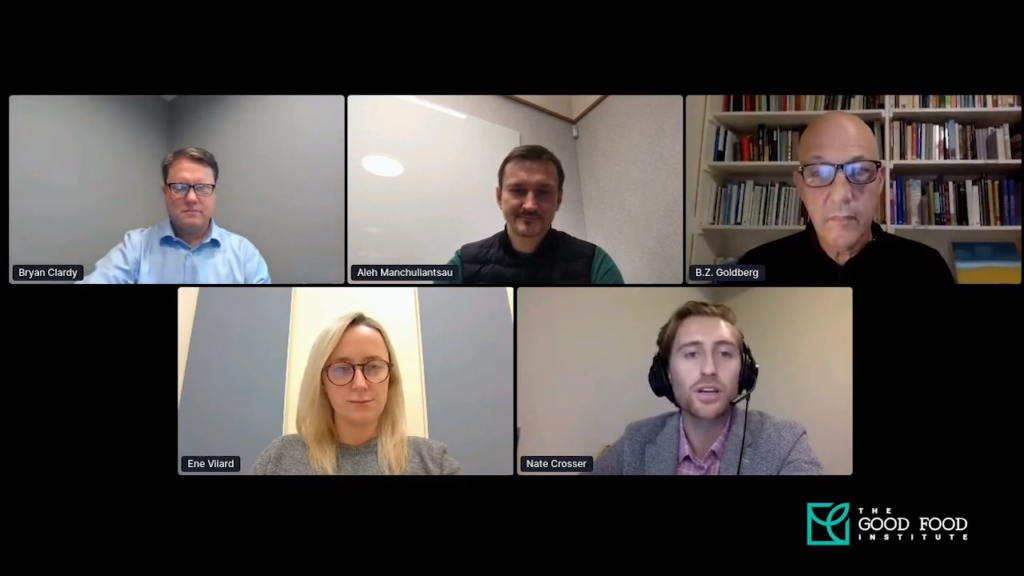
Explore research opportunities in fermentation
-
Cultivated
-
Fermentation
-
Plant-Based
Optimizing fat profiles for nutritional and sensory properties
Because alternative meat’s fat content and fatty acid profile can be more easily controlled than conventional meat’s, there is an opportunity to alter fat content for nutritional benefits. Additional research…
-
Cultivated
-
Fermentation
-
Plant-Based
Life Cycle Assessment for alternative seafood relative to conventional fishing and aquaculture
To date, no robust environmental assessments have been conducted to compare alternative seafood to its conventional counterparts. An open-access, quantitative analysis of the relative environmental impacts of alternative seafood will…
-
Cultivated
-
Fermentation
-
Plant-Based
Repurposing and retrofitting facilities for use in alternative protein manufacturing
The manufacturing capacity for rapid and cost-effective scale-up of alternative protein production is a current constraint on the growth of the industry. Repurposing and retrofitting stranded or underutilized assets such…
Meet the author
Liz Specht, Ph.D.
DIRECTOR OF SCIENCE AND TECHNOLOGY
Liz Specht oversees GFI’s Science and Technology department to build a roadmap for accelerating alternative protein research while empowering scientists to execute on this vision.
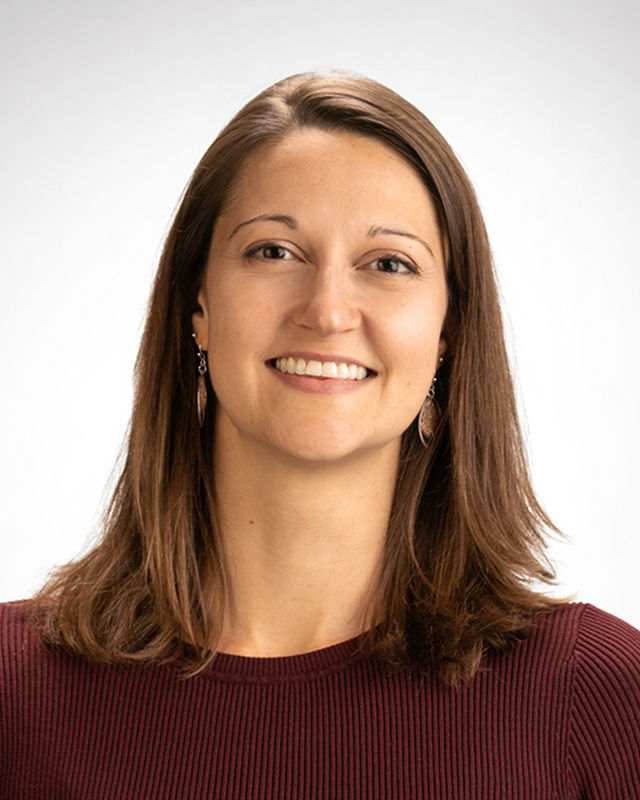
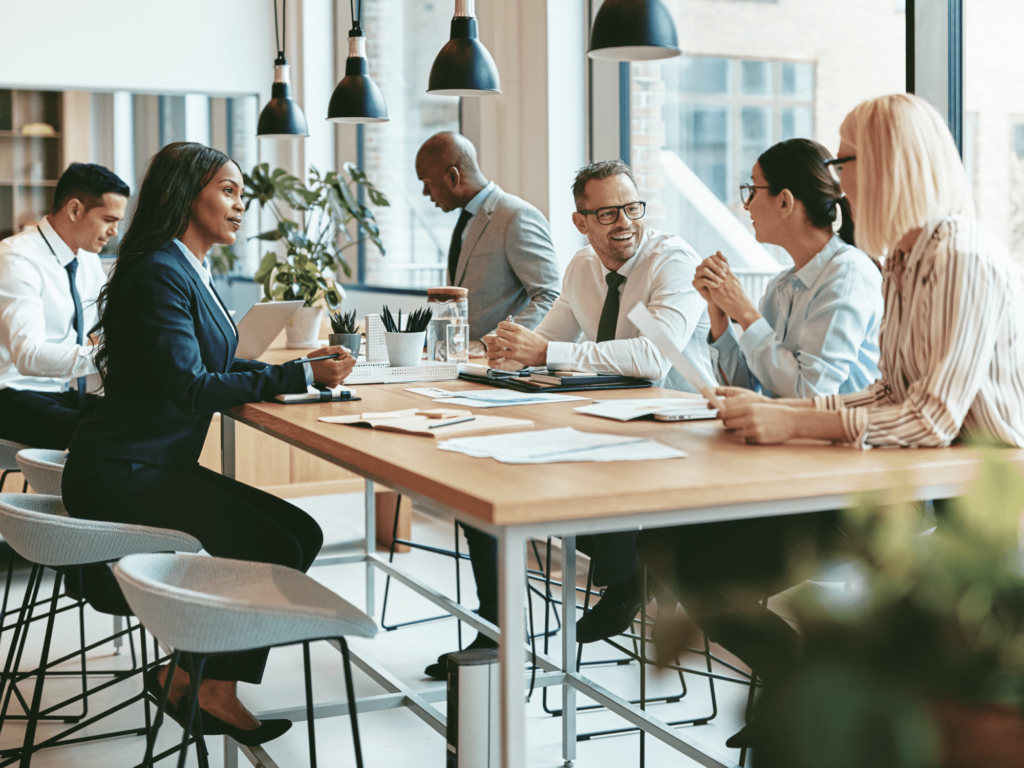
Meet our science & technology advisory board
Our science & technology advisory board expands our department’s expert technical capacity by supporting team initiatives, sharing ecosystem insights, and providing strategic feedback on our team’s future activities.
Header photo courtesy of Meati